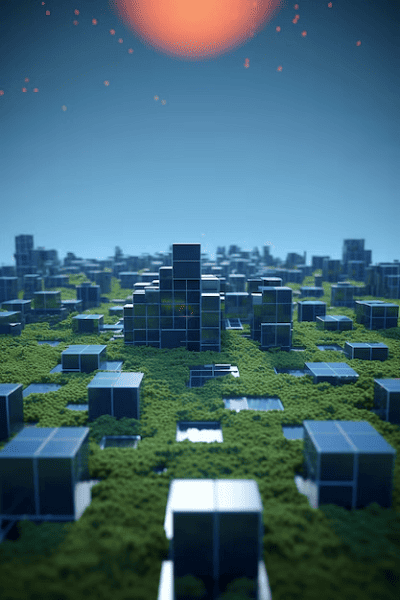
Sustainable technology of 4140 ?
4140 steel is a versatile alloy steel that is widely used in various industries due to its excellent combination of strength, toughness, and wear resistance. While the production of any steel involves environmental considerations, there are ways to make the use of 4140 steel more sustainable:
1. Recycling: 4140 steel is readily recyclable. Recycling steel significantly reduces the need for virgin resources and lowers energy consumption compared to producing steel from raw materials.
2. Efficient Manufacturing Processes:
- Near-net shape manufacturing: Techniques like forging or casting can be used to produce parts closer to their final shape, reducing material waste and machining time.
- Optimized machining: Employing efficient machining practices, such as using high-speed machining techniques, appropriate cutting tools, and optimizing cutting parameters, can minimize material removal and energy consumption.
- Heat treatment optimization: Precisely controlling heat treatment processes can enhance the material properties of 4140 steel, extending its service life and reducing the need for frequent replacements.
3. Extending Service Life:
- Proper maintenance: Regular inspection and maintenance of components made from 4140 steel can help identify and address potential issues early on, preventing premature failure and extending their lifespan.
- Surface treatments: Applying appropriate surface treatments, such as coatings or hardening processes, can improve the wear and corrosion resistance of 4140 steel, further prolonging its use.
4. Responsible Sourcing:
- Sustainable steel mills: Choosing suppliers that prioritize sustainable practices, such as using renewable energy sources, reducing emissions, and managing waste responsibly, can contribute to the overall sustainability of 4140 steel usage.
5. Design for Durability:
- Robust design: Designing components with 4140 steel that are robust and durable can minimize the risk of failure and extend their service life, reducing the need for replacements.
By incorporating these sustainable practices throughout the lifecycle of 4140 steel, from production to end-of-life management, we can minimize its environmental impact and contribute to a more sustainable future.
What is Sustainable technology of 4140 ?
This is for informational purposes only. For medical advice or diagnosis, consult a professional.
Sustainable technology of 4140 steel encompasses practices and innovations that minimize the environmental impact throughout the steel’s lifecycle. Here’s a breakdown:
1. Production:
- Electric Arc Furnaces (EAFs): EAFs use electricity to melt recycled steel, reducing reliance on coal and lowering carbon emissions compared to traditional Basic Oxygen Furnaces.
- Energy Efficiency: Steel mills are adopting energy-efficient technologies like waste heat recovery and process optimization to reduce energy consumption.
2. Manufacturing:
- Near-net Shape Manufacturing: Techniques like forging and casting produce parts closer to their final shape, minimizing material waste and machining time.
- Optimized Machining: Efficient machining practices, such as high-speed machining and optimized cutting tools, minimize material removal and energy use.
3. Extending Service Life:
- Surface Treatments: Coatings and hardening processes improve wear and corrosion resistance, extending the lifespan of 4140 steel components.
- Proper Maintenance: Regular inspection and maintenance prevent premature failure, reducing the need for replacements.
4. End-of-Life:
- Recycling: 4140 steel is readily recyclable. Recycling steel reduces the need for virgin resources and lowers energy consumption compared to producing steel from raw materials.
5. Design:
- Design for Durability: Robust designs minimize the risk of failure and extend the service life of components.
- Lightweighting: Where possible, optimizing designs to use less material reduces overall environmental impact.
Examples of Sustainable Technologies:
- Additive Manufacturing (3D printing): Can create complex shapes with minimal waste, potentially reducing the amount of 4140 steel needed.
- Laser Surface Treatment: A precise method for hardening steel surfaces, potentially extending component life with minimal energy input.
By incorporating these sustainable practices throughout the lifecycle of 4140 steel, we can minimize its environmental impact and contribute to a more sustainable future.
Who is required Sustainable technology of 4140 ?
This is for informational purposes only. For medical advice or diagnosis, consult a professional.
Sustainable technology of 4140 steel is required by anyone involved in the lifecycle of this material, from production to end-of-life management. This includes:
- Steel Manufacturers: Implementing sustainable practices like using electric arc furnaces, optimizing energy efficiency, and minimizing waste.
- Manufacturers of 4140 Steel Components: Employing near-net shape manufacturing, optimizing machining processes, and extending service life through surface treatments and proper maintenance.
- Engineers and Designers: Designing for durability, lightweighting where possible, and considering end-of-life recyclability in their designs.
- Consumers: Choosing products made from sustainably sourced 4140 steel and properly maintaining and recycling these products.
- Policymakers: Implementing regulations and incentives that encourage sustainable practices in the steel industry.
By everyone playing their part, we can minimize the environmental impact of 4140 steel and contribute to a more sustainable future.
When is required Sustainable technology of 4140 ?
This is for informational purposes only. For medical advice or diagnosis, consult a professional.
Sustainable technology of 4140 steel is required throughout the lifecycle of the material, from production to end-of-life management. Here’s a breakdown:
- Steel Manufacturers: Implementing sustainable practices like using electric arc furnaces, optimizing energy efficiency, and minimizing waste.
- Manufacturers of 4140 Steel Components: Employing near-net shape manufacturing, optimizing machining processes, and extending service life through surface treatments and proper maintenance.
- Engineers and Designers: Designing for durability, lightweighting where possible, and considering end-of-life recyclability in their designs.
- Consumers: Choosing products made from sustainably sourced 4140 steel and properly maintaining and recycling these products.
- Policymakers: Implementing regulations and incentives that encourage sustainable practices in the steel industry.
By everyone playing their part, we can minimize the environmental impact of 4140 steel and contribute to a more sustainable future.
COURTESY : Voith Group
Where is required Sustainable technology of 4140 ?
This is for informational purposes only. For medical advice or diagnosis, consult a professional.
Sustainable technology of 4140 steel is required wherever this material is used. This includes:
- Steel Mills: Implementing sustainable practices like using electric arc furnaces, optimizing energy efficiency, and minimizing waste.
- Manufacturing Facilities: Employing near-net shape manufacturing, optimizing machining processes, and extending service life through surface treatments and proper maintenance.
- Engineering and Design Firms: Designing for durability, lightweighting where possible, and considering end-of-life recyclability in their designs.
- Consumers: Choosing products made from sustainably sourced 4140 steel and properly maintaining and recycling these products.
- Government Agencies: Implementing regulations and incentives that encourage sustainable practices in the steel industry.
By everyone playing their part, we can minimize the environmental impact of 4140 steel and contribute to a more sustainable future.
How is required Sustainable technology of 4140 ?
This is for informational purposes only. For medical advice or diagnosis, consult a professional.
Sustainable technology of 4140 steel is required to be implemented through a multi-faceted approach involving all stakeholders in the material’s lifecycle. Here’s how:
- Steel Manufacturers: Adopting sustainable practices like using electric arc furnaces, optimizing energy efficiency, and minimizing waste through process improvements and closed-loop systems.
- Manufacturers of 4140 Steel Components: Employing near-net shape manufacturing to reduce material waste, optimizing machining processes to minimize energy consumption, and extending service life through surface treatments and proper maintenance.
- Engineers and Designers: Designing for durability, lightweighting where possible, and considering end-of-life recyclability in their designs to minimize the overall environmental impact of 4140 steel components.
- Consumers: Making informed choices by selecting products made from sustainably sourced 4140 steel, properly maintaining these products to extend their lifespan, and ensuring they are recycled at the end of their use.
- Policymakers: Implementing regulations and incentives that encourage sustainable practices in the steel industry, such as carbon pricing, recycled content mandates, and support for research and development of sustainable steelmaking technologies.
By everyone playing their part, we can collectively drive the adoption of sustainable technology for 4140 steel, minimizing its environmental impact and contributing to a more sustainable future.
Case study is Sustainable technology of 4140 ?
Let’s explore a hypothetical case study demonstrating the application of sustainable technology with 4140 steel. Since specific real-world case studies with detailed data are often proprietary, this will be a realistic scenario illustrating the concepts.
Case Study: Sustainable Manufacturing of a 4140 Steel Gear for Heavy Machinery
Product: A large gear used in the drivetrain of heavy construction equipment. These gears are traditionally made from 4140 steel due to its strength and toughness.
Challenge: The manufacturing process has high material waste, energy consumption, and transportation costs. The gears also have a limited lifespan due to wear.
Sustainable Solution:
- Near-Net Shape Forging: Instead of machining the gear from a large block of steel (resulting in significant waste), the gear is forged to near-net shape. This significantly reduces the amount of raw material needed and minimizes subsequent machining. This also improves the grain structure of the steel, enhancing its strength and fatigue resistance.
- Optimized Machining: Precision machining techniques are employed to remove only the necessary material to achieve the final gear dimensions. This includes using high-efficiency cutting tools and optimizing cutting parameters to reduce energy consumption and further minimize waste. Coolants and lubricants are used judiciously and recycled.
- Induction Hardening: Instead of carburizing or through-hardening the entire gear, which is energy-intensive, induction hardening is used to selectively harden only the gear teeth. This significantly reduces energy consumption and extends the gear’s lifespan by improving wear resistance.
- Surface Coating: A wear-resistant coating (e.g., a PVD coating) is applied to the gear teeth to further enhance their durability and extend the gear’s service life. This reduces the frequency of replacements, saving material and energy in the long run.
- Closed-Loop Recycling: Scrap 4140 steel from the forging and machining processes is collected and recycled back into the steelmaking process, reducing the demand for virgin raw materials.
- Transportation Optimization: The manufacturing facility is located closer to the raw material source and the end-user (heavy machinery manufacturer) to minimize transportation distances and associated fuel consumption and emissions.
Results:
- Material Savings: Near-net shape forging reduces raw material consumption by an estimated 40-50%.
- Energy Reduction: Optimized machining and induction hardening reduce energy consumption during manufacturing by an estimated 30-40%.
- Extended Lifespan: The combination of induction hardening and surface coating extends the gear’s service life by an estimated 50-75%, reducing the need for replacements.
- Waste Reduction: Closed-loop recycling minimizes waste and reduces the environmental impact of disposal.
- Reduced Transportation Costs and Emissions: Optimized transportation logistics contribute to lower costs and reduced greenhouse gas emissions.
Conclusion:
This case study demonstrates how a combination of sustainable technologies can be applied throughout the lifecycle of a 4140 steel component to significantly reduce its environmental impact while also improving performance and potentially lowering overall costs. This approach highlights the importance of considering sustainability at every stage, from material sourcing and manufacturing to end-of-life management.
COURTESY : NPTEL-NOC IITM
White paper on Sustainable technology of 4140 ?
White Paper: Sustainable Practices for 4140 Alloy Steel: A Lifecycle Approach
Abstract:
4140 alloy steel, prized for its strength, toughness, and wear resistance, finds widespread application across diverse industries. However, its production and processing carry environmental implications. This white paper explores sustainable technologies and practices applicable throughout the 4140 steel lifecycle, from raw material sourcing and manufacturing to end-of-life management, aiming to minimize environmental impact and promote resource efficiency.
1. Introduction:
4140 steel, a medium-carbon, low-alloy steel, is ubiquitous in applications demanding high strength and toughness, including gears, shafts, fasteners, and structural components. While its performance characteristics are advantageous, traditional manufacturing processes can be resource-intensive and generate waste. This necessitates a shift towards sustainable practices that encompass the entire lifecycle of 4140 steel.
2. Sustainable Production of 4140 Steel:
- Electric Arc Furnaces (EAFs): Transitioning from Basic Oxygen Furnaces (BOFs) to EAFs, which utilize electricity to melt scrap steel, significantly reduces reliance on coal and lowers carbon emissions. Promoting the use of renewable energy sources to power EAFs further enhances sustainability.
- Energy Efficiency in Steel Mills: Implementing energy-efficient technologies within steel mills, such as waste heat recovery systems, process optimization, and advanced control systems, minimizes energy consumption and reduces greenhouse gas emissions.
- Responsible Sourcing of Raw Materials: Emphasizing responsible sourcing of raw materials, including iron ore and alloying elements, ensures environmentally sound mining practices and minimizes ecological disruption.
3. Sustainable Manufacturing of 4140 Steel Components:
- Near-Net Shape Manufacturing: Employing near-net shape manufacturing techniques like forging, casting, or powder metallurgy significantly reduces material waste compared to traditional machining from large stock. This also reduces energy consumption associated with material removal.
- Optimized Machining Processes: Implementing efficient machining practices, including high-speed machining, optimized cutting tools, and minimal lubrication strategies, minimizes material removal, energy consumption, and waste generation.
- Surface Treatments for Enhanced Durability: Applying surface treatments such as coatings (e.g., PVD, nitriding) or laser hardening enhances wear and corrosion resistance, extending the service life of 4140 steel components and reducing the need for frequent replacements.
- Additive Manufacturing (3D Printing): Exploring the application of additive manufacturing for specific 4140 steel components enables the creation of complex geometries with minimal material waste, potentially revolutionizing manufacturing processes.
4. Extending the Service Life of 4140 Steel Components:
- Preventive Maintenance: Implementing robust preventive maintenance programs, including regular inspections, lubrication, and timely repairs, extends the operational life of 4140 steel components, reducing the demand for replacements.
- Refurbishment and Reconditioning: Exploring opportunities for refurbishing and reconditioning worn or damaged 4140 steel components, rather than discarding them, can significantly reduce material consumption and environmental impact.
5. End-of-Life Management and Recycling:
- Closed-Loop Recycling: Establishing closed-loop recycling systems for 4140 steel scrap generated during manufacturing and at the end of product life ensures that valuable material is recovered and reused, minimizing the need for virgin raw materials.
- Design for Recyclability: Designing 4140 steel components with recyclability in mind, including ease of disassembly and material separation, facilitates efficient recycling processes.
6. Design Considerations for Sustainability:
- Design for Durability: Designing 4140 steel components for durability and longevity minimizes the risk of failure and extends their service life, reducing the need for replacements.
- Lightweighting: Optimizing designs to reduce the amount of 4140 steel required for a given application minimizes material consumption and can improve energy efficiency in transportation applications.
7. Conclusion:
Achieving sustainability with 4140 steel requires a holistic approach that considers the entire lifecycle of the material. By implementing the sustainable technologies and practices outlined in this white paper, stakeholders across the value chain, from steel producers and manufacturers to end-users and policymakers, can collectively contribute to minimizing the environmental impact of 4140 steel and promoting a more circular economy. Further research and development in areas like advanced manufacturing techniques, surface treatments, and recycling technologies will be crucial for achieving long-term sustainability goals.
Industrial application of Sustainable technology of 4140 ?
This is for informational purposes only. For medical advice or diagnosis, consult a professional.
Sustainable technology of 4140 steel is being applied across various industries to minimize environmental impact and promote resource efficiency. Here are some key industrial applications:
- Aerospace: Lightweighting initiatives in aircraft design are driving the use of 4140 steel in components like landing gear and structural parts. This reduces fuel consumption and emissions.
- Automotive: 4140 steel is used in gears, shafts, and other critical components. Sustainable manufacturing practices like near-net shape forging and optimized machining reduce material waste and energy consumption.
- Oil and Gas: 4140 steel is used in downhole tools and drilling equipment. Surface treatments that enhance wear and corrosion resistance extend the lifespan of these components, reducing the need for replacements.
- Manufacturing: 4140 steel is used in molds, dies, and tooling. Additive manufacturing (3D printing) enables the creation of complex shapes with minimal material waste, revolutionizing manufacturing processes.
- Renewable Energy: 4140 steel is used in wind turbine components. Sustainable practices in manufacturing and maintenance contribute to the overall sustainability of renewable energy technologies.
By implementing sustainable technologies across these diverse industrial applications, we can minimize the environmental impact of 4140 steel and contribute to a more sustainable future.
Research and development of Sustainable technology of 4140 ?
This is for informational purposes only. For medical advice or diagnosis, consult a professional.
Research and development (R&D) plays a crucial role in advancing sustainable technology for 4140 steel. Here are some key areas of focus:
- Advanced Manufacturing Processes:
- Additive Manufacturing (3D printing): Developing techniques to produce high-density, defect-free 4140 steel parts using additive manufacturing can significantly reduce material waste and enable the creation of complex geometries.
- Near-Net Shape Manufacturing: Improving existing near-net shape processes like forging and casting to further minimize material removal and energy consumption.
- Surface Treatments:
- Environmentally Friendly Coatings: Researching and developing new coatings that provide enhanced wear and corrosion resistance while minimizing environmental impact.
- Laser Surface Treatment: Optimizing laser surface treatment techniques to selectively harden 4140 steel components with minimal energy input and precise control.
- Recycling Technologies:
- Efficient Recycling Processes: Developing innovative recycling technologies to improve the recovery and reuse of 4140 steel scrap, including techniques for separating and purifying alloying elements.
- Design for Recyclability: Researching design principles that facilitate the disassembly and separation of 4140 steel components for easier recycling.
- Life Cycle Assessment:
- Data Collection and Analysis: Conducting comprehensive life cycle assessments to quantify the environmental impacts of 4140 steel production, manufacturing, and end-of-life management.
- Optimization Strategies: Using life cycle assessment data to identify areas for improvement and develop strategies to minimize environmental impacts throughout the 4140 steel lifecycle.
- Sustainable Steelmaking:
- Electric Arc Furnace Technology: Improving EAF technology to further reduce carbon emissions and increase the use of renewable energy sources.
- Alternative Ironmaking Processes: Exploring alternative ironmaking processes that reduce reliance on fossil fuels and lower greenhouse gas emissions.
By investing in R&D across these areas, we can accelerate the development and adoption of sustainable technologies for 4140 steel, minimizing its environmental impact and promoting a circular economy.
COURTESY : Bernard Marr
References
- ^ Jump up to:a b c Kutscher, Milford & Kreith 2019, pp. 5–6.
- ^ Zhang, Wei; Li, Binshuai; Xue, Rui; Wang, Chengcheng; Cao, Wei (2021). “A systematic bibliometric review of clean energy transition: Implications for low-carbon development”. PLOS ONE. 16 (12): e0261091. Bibcode:2021PLoSO..1661091Z. doi:10.1371/journal.pone.0261091. PMC 8641874. PMID 34860855.
- ^ United Nations Development Programme 2016, p. 5.
- ^ “Definitions: energy, sustainability and the future”. OpenLearn. The Open University. Archived from the original on 27 January 2021. Retrieved 30 December 2020.
- ^ Golus̆in, Popov & Dodić 2013, p. 8.
- ^ Jump up to:a b c d Hammond, Geoffrey P.; Jones, Craig I. “Sustainability criteria for energy resources and technologies”. In Galarraga, González-Eguino & Markandya (2011), pp. 21–47.
- ^ Jump up to:a b c d UNECE 2020, pp. 3–4.
- ^ Gunnarsdottir, I.; Davidsdottir, B.; Worrell, E.; Sigurgeirsdottir, S. (May 2021). “Sustainable energy development: History of the concept and emerging themes”. Renewable and Sustainable Energy Reviews. 141: 110770. Bibcode:2021RSERv.14110770G. doi:10.1016/j.rser.2021.110770. hdl:1874/411740.
- ^ Kutscher, Milford & Kreith 2019, pp. 1–2.
- ^ Vera, Ivan; Langlois, Lucille (2007). “Energy indicators for sustainable development”. Energy. 32 (6): 875–882. Bibcode:2007Ene….32..875V. doi:10.1016/j.energy.2006.08.006.
- ^ Kutscher, Milford & Kreith 2019, pp. 3–5.
- ^ Ritchie, Hannah; Roser, Max (2021). “What are the safest and cleanest sources of energy?”. Our World in Data. Archived from the original on 15 January 2024. Data sources: Markandya & Wilkinson (2007); UNSCEAR (2008; 2018); Sovacool et al. (2016); IPCC AR5 (2014); Pehl et al. (2017); Ember Energy (2021).
- ^ Jump up to:a b United Nations Environment Programme 2019, p. 46.
- ^ “Global Historical Emissions”. Climate Watch. Archived from the original on 4 June 2021. Retrieved 19 August 2021.
- ^ Ge, Mengpin; Friedrich, Johannes; Vigna, Leandro (August 2021). “4 Charts Explain Greenhouse Gas Emissions by Countries and Sectors”. World Resources Institute. Archived from the original on 19 August 2021. Retrieved 19 August 2021.
- ^ “The Paris Agreement”. United Nations Framework Convention on Climate Change. Archived from the original on 19 March 2021. Retrieved 18 September 2021.
- ^ Watts, Nick; Amann, Markus; Arnell, Nigel; Ayeb-Karlsson, Sonja; et al. (2021). “The 2020 report of The Lancet Countdown on health and climate change: responding to converging crises” (PDF). The Lancet. 397 (10269): 151. Bibcode:2021Lanc..397..129W. doi:10.1016/S0140-6736(20)32290-X. PMC 7616803. PMID 33278353.
- ^ “Every breath you take: The staggering, true cost of air pollution”. United Nations Development Programme. 4 June 2019. Archived from the original on 20 April 2021. Retrieved 4 May 2021.
- ^ “New WHO Global Air Quality Guidelines aim to save millions of lives from air pollution”. World Health Organization. 22 September 2021. Archived from the original on 23 September 2021. Retrieved 16 October 2021.
- ^ “Acid Rain and Water”. United States Geological Survey. Archived from the original on 27 June 2021. Retrieved 14 October 2021.
- ^ Jump up to:a b World Health Organization 2018, p. 16.
- ^ “Ambient (outdoor) air pollution”. World Health Organization. 22 September 2021. Archived from the original on 8 October 2021. Retrieved 22 October 2021.
- ^ Ritchie, Hannah; Roser, Max (2019). “Access to Energy”. Our World in Data. Archived from the original on 1 April 2021. Retrieved 1 April 2021.
- ^ Jump up to:a b World Health Organization 2016, pp. vii–xiv.
- ^ Soysal & Soysal 2020, p. 118.
- ^ Soysal & Soysal 2020, pp. 470–472.
- ^ Tester et al. 2012, p. 504.
- ^ Kessides, Ioannis N.; Toman, Michael (28 July 2011). “The Global Energy Challenge”. World Bank. Archived from the original on 25 July 2019. Retrieved 27 September 2019.
- ^ Morris et al. 2015, pp. 24–27.
- ^ “Access to clean cooking”. SDG7: Data and Projections. IEA. October 2020. Archived from the original on 6 December 2019. Retrieved 31 March 2021.
- ^ IEA 2021, p. 167.
- ^ Sarkodie, Samuel Asumadu (20 July 2022). “Winners and losers of energy sustainability—Global assessment of the Sustainable Development Goals”. Science of the Total Environment. 831. 154945. Bibcode:2022ScTEn.83154945S. doi:10.1016/j.scitotenv.2022.154945. hdl:11250/3023660. PMID 35367559.
- ^ Deputy Secretary-General (6 June 2018). “Sustainable Development Goal 7 on Reliable, Modern Energy ‘Golden Thread’ Linking All Other Targets, Deputy-Secretary-General Tells High-Level Panel” (Press release). United Nations. Archived from the original on 17 May 2021. Retrieved 19 March 2021.
- ^ Jump up to:a b “Goal 7: Ensure access to affordable, reliable, sustainable and modern energy for all”. SDG Tracker. Archived from the original on 2 February 2021. Retrieved 12 March 2021.
- ^ “Energy use per person”. Our World in Data. Archived from the original on 28 November 2020. Retrieved 16 July 2021.
- ^ “Europe 2030: Energy saving to become “first fuel””. EU Science Hub. European Commission. 25 February 2016. Archived from the original on 18 September 2021. Retrieved 18 September 2021.
- ^ Motherway, Brian (19 December 2019). “Energy efficiency is the first fuel, and demand for it needs to grow”. IEA. Archived from the original on 18 September 2021. Retrieved 18 September 2021.
- ^ “Energy Efficiency 2018: Analysis and outlooks to 2040”. IEA. October 2018. Archived from the original on 29 September 2020.
- ^ Fernandez Pales, Araceli; Bouckaert, Stéphanie; Abergel, Thibaut; Goodson, Timothy (10 June 2021). “Net zero by 2050 hinges on a global push to increase energy efficiency”. IEA. Archived from the original on 20 July 2021. Retrieved 19 July 2021.
- ^ Jump up to:a b IEA 2021, pp. 68–69.
- ^ Mundaca, Luis; Ürge-Vorsatz, Diana; Wilson, Charlie (February 2019). “Demand-side approaches for limiting global warming to 1.5 °C”. Energy Efficiency. 12 (2): 343–362. Bibcode:2019EnEff..12..343M. doi:10.1007/s12053-018-9722-9.
- ^ Jump up to:a b IEA, IRENA, United Nations Statistics Division, World Bank, World Health Organization 2021, p. 12.
- ^ Jump up to:a b IEA, IRENA, United Nations Statistics Division, World Bank, World Health Organization 2021, p. 11.
- ^ Brockway, Paul E.; Sorrell, Steve; Semieniuk, Gregor; Heun, Matthew Kuperus; Court, Victor (May 2021). “Energy efficiency and economy-wide rebound effects: A review of the evidence and its implications”. Renewable and Sustainable Energy Reviews. 141: 110781. Bibcode:2021RSERv.14110781B. doi:10.1016/j.rser.2021.110781.
- ^ “Energy Efficiency 2019”. IEA. November 2019. Archived from the original on 13 October 2020. Retrieved 21 September 2020.
- ^ Bond, Kingsmill; Butler-Sloss, Sam; Lovins, Amory; Speelman, Laurens; Topping, Nigel (13 June 2023). “Report / 2023 / X-Change: Electricity / On track for disruption”. Rocky Mountain Institute. Archived from the original on 13 July 2023.
- ^ Source for data beginning in 2017: “Renewable Energy Market Update Outlook for 2023 and 2024” (PDF). International Energy Agency. June 2023. p. 19. Archived (PDF) from the original on 11 July 2023.
IEA. CC BY 4.0.
● Source for data through 2016: “Renewable Energy Market Update / Outlook for 2021 and 2022” (PDF). IEA.org. International Energy Agency. May 2021. p. 8. Archived (PDF) from the original on 25 March 2023.IEA. Licence: CC BY 4.0
- ^ “World Energy Investment 2023 / Overview and key findings”. International Energy Agency (IEA). 25 May 2023. Archived from the original on 31 May 2023.
Global energy investment in clean energy and in fossil fuels, 2015-2023 (chart)
— From pages 8 and 12 of World Energy Investment 2023 (archive). - ^ IEA 2007, p. 3.
- ^ Santangeli, Andrea; Toivonen, Tuuli; Pouzols, Federico Montesino; Pogson, Mark; Hastings, Astley; Smith, Pete; Moilanen, Atte (September 2016). “Global change synergies and trade-offs between renewable energy and biodiversity”. GCB Bioenergy. 8 (5): 941–951. Bibcode:2016GCBBi…8..941S. doi:10.1111/gcbb.12299. hdl:2164/6138.
- ^ Rehbein, Jose A.; Watson, James E. M.; Lane, Joe L.; Sonter, Laura J.; Venter, Oscar; Atkinson, Scott C.; Allan, James R. (May 2020). “Renewable energy development threatens many globally important biodiversity areas”. Global Change Biology. 26 (5): 3040–3051. Bibcode:2020GCBio..26.3040R. doi:10.1111/gcb.15067. PMID 32133726.
- ^ Ritchie, Hannah (2019). “Renewable Energy”. Our World in Data. Archived from the original on 4 August 2020. Retrieved 31 July 2020.
- ^ Renewables 2020 Analysis and forecast to 2025 (PDF) (Report). IEA. 2020. p. 12. Archived from the original on 26 April 2021.
- ^ “Access to electricity”. SDG7: Data and Projections. IEA. 2020. Archived from the original on 13 May 2021. Retrieved 5 May 2021.
- ^ “Infrastructure Solutions: The power of purchase agreements”. European Investment Bank. Retrieved 1 September 2022.
- ^ “Renewable Power – Analysis”. IEA. Retrieved 1 September 2022.
- ^ “Global Electricity Review 2022”. Ember. 29 March 2022. Retrieved 1 September 2022.
- ^ “Renewable Energy and Electricity | Sustainable Energy | Renewable Energy – World Nuclear Association”. world-nuclear.org. Retrieved 1 September 2022.
- ^ Jump up to:a b IEA (2022), Renewables 2022, IEA, Paris https://www.iea.org/reports/renewables-2022, License: CC BY 4.0
- ^ Soysal & Soysal 2020, p. 406.
- ^ Jump up to:a b c “Wind & Solar Share in Electricity Production Data”. Global Energy Statistical Yearbook 2021. Enerdata. Archived from the original on 19 July 2019. Retrieved 13 June 2021.
- ^ Kutscher, Milford & Kreith 2019, pp. 34–35.
- ^ Jump up to:a b “Levelized Cost of Energy and of Storage”. Lazard. 19 October 2020. Archived from the original on 25 February 2021. Retrieved 26 February 2021.
- ^ Victoria, Marta; Haegel, Nancy; Peters, Ian Marius; Sinton, Ron; Jäger-Waldau, Arnulf; del Cañizo, Carlos; Breyer, Christian; Stocks, Matthew; Blakers, Andrew; Kaizuka, Izumi; Komoto, Keiichi; Smets, Arno (May 2021). “Solar photovoltaics is ready to power a sustainable future”. Joule. 5 (5): 1041–1056. Bibcode:2021Joule…5.1041V. doi:10.1016/j.joule.2021.03.005.
- ^ IRENA 2021, pp. 19, 22.
- ^ Goetz, Katelyn P.; Taylor, Alexander D.; Hofstetter, Yvonne J.; Vaynzof, Yana (13 January 2021). “Sustainability in Perovskite Solar Cells”. ACS Applied Materials & Interfaces. 13 (1): 1–17. doi:10.1021/acsami.0c17269. PMID 33372760.
- ^ Xu, Yan; Li, Jinhui; Tan, Quanyin; Peters, Anesia Lauren; Yang, Congren (May 2018). “Global status of recycling waste solar panels: A review”. Waste Management. 75: 450–458. Bibcode:2018WaMan..75..450X. doi:10.1016/j.wasman.2018.01.036. PMID 29472153.
- ^ Tian, Xueyu; Stranks, Samuel D.; You, Fengqi (31 July 2020). “Life cycle energy use and environmental implications of high-performance perovskite tandem solar cells”. Science Advances. 6 (31): eabb0055. Bibcode:2020SciA….6…55T. doi:10.1126/sciadv.abb0055. PMC 7399695. PMID 32789177.
- ^ Kutscher, Milford & Kreith 2019, pp. 35–36.
- ^ “Solar energy”. International Renewable Energy Agency. Archived from the original on 13 May 2021. Retrieved 5 June 2021.
- ^ REN21 2020, p. 124.
- ^ Soysal & Soysal 2020, p. 366.
- ^ “What are the advantages and disadvantages of offshore wind farms?”. American Geosciences Institute. 12 May 2016. Archived from the original on 18 September 2021. Retrieved 18 September 2021.
- ^ Szarka 2007, p. 176.
- ^ Wang, Shifeng; Wang, Sicong (September 2015). “Impacts of wind energy on environment: A review”. Renewable and Sustainable Energy Reviews. 49: 437–443. Bibcode:2015RSERv..49..437W. doi:10.1016/j.rser.2015.04.137.
- ^ Soysal & Soysal 2020, p. 215.
- ^ Soysal & Soysal 2020, p. 213.
- ^ Huang, Yu-Fong; Gan, Xing-Jia; Chiueh, Pei-Te (March 2017). “Life cycle assessment and net energy analysis of offshore wind power systems”. Renewable Energy. 102: 98–106. Bibcode:2017REne..102…98H. doi:10.1016/j.renene.2016.10.050.
- ^ Belton, Padraig (7 February 2020). “What happens to all the old wind turbines?”. BBC. Archived from the original on 23 February 2021. Retrieved 27 February 2021.
- ^ Smil 2017b, p. 286.
- ^ REN21 2021, p. 21.
- ^ Jump up to:a b c Moran, Emilio F.; Lopez, Maria Claudia; Moore, Nathan; Müller, Norbert; Hyndman, David W. (20 November 2018). “Sustainable hydropower in the 21st century”. Proceedings of the National Academy of Sciences. 115 (47): 11891–11898. Bibcode:2018PNAS..11511891M. doi:10.1073/pnas.1809426115. PMC 6255148. PMID 30397145.
- ^ Kumar, A.; Schei, T.; Ahenkorah, A.; Caceres Rodriguez, R. et al. “Hydropower“. In IPCC (2011), pp. 451, 462, 488.
- ^ Jump up to:a b c Schlömer, S.; Bruckner, T.; Fulton, L.; Hertwich, E. et al. “Annex III: Technology-specific cost and performance parameters“. In IPCC (2014), p. 1335.
- ^ Almeida, Rafael M.; Shi, Qinru; Gomes-Selman, Jonathan M.; Wu, Xiaojian; Xue, Yexiang; Angarita, Hector; Barros, Nathan; Forsberg, Bruce R.; García-Villacorta, Roosevelt; Hamilton, Stephen K.; Melack, John M.; Montoya, Mariana; Perez, Guillaume; Sethi, Suresh A.; Gomes, Carla P.; Flecker, Alexander S. (19 September 2019). “Reducing greenhouse gas emissions of Amazon hydropower with strategic dam planning”. Nature Communications. 10 (1): 4281. doi:10.1038/s41467-019-12179-5. PMC 6753097. PMID 31537792.
- ^ László, Erika (1981). “Geothermal Energy: An Old Ally”. Ambio. 10 (5): 248–249. JSTOR 4312703.
- ^ REN21 2020, p. 97.
- ^ “Geothermal Energy Information and Facts”. National Geographic. 19 October 2009. Archived from the original on 8 August 2021. Retrieved 8 August 2021.
- ^ Jump up to:a b Ritchie, Hannah; Roser, Max (2020). “Energy mix”. Our World in Data. Archived from the original on 2 July 2021. Retrieved 9 July 2021.
- ^ Soysal & Soysal 2020, pp. 222, 228.
- ^ Soysal & Soysal 2020, pp. 228–229.
- ^ “Biomass explained”. US Energy Information Administration. 8 June 2021. Archived from the original on 15 September 2021. Retrieved 13 September 2021.
- ^ Kopetz, Heinz (7 February 2013). “Build a biomass energy market”. Nature. 494 (7435): 29–31. doi:10.1038/494029a. PMID 23389528.
- ^ Demirbas, Ayhan (August 2008). “Biofuels sources, biofuel policy, biofuel economy and global biofuel projections”. Energy Conversion and Management. 49 (8): 2106–2116. Bibcode:2008ECM….49.2106D. doi:10.1016/j.enconman.2008.02.020.
- ^ Jump up to:a b c Correa, Diego F.; Beyer, Hawthorne L.; Fargione, Joseph E.; Hill, Jason D.; Possingham, Hugh P.; Thomas-Hall, Skye R.; Schenk, Peer M. (June 2019). “Towards the implementation of sustainable biofuel production systems”. Renewable and Sustainable Energy Reviews. 107: 250–263. Bibcode:2019RSERv.107..250C. doi:10.1016/j.rser.2019.03.005.
- ^ Daley, Jason (24 April 2018). “The EPA Declared That Burning Wood Is Carbon Neutral. It’s Actually a Lot More Complicated”. Smithsonian Magazine. Archived from the original on 30 June 2021. Retrieved 14 September 2021.
- ^ Tester et al. 2012, p. 512.
- ^ Jump up to:a b Smil 2017a, p. 162.
- ^ World Health Organization 2016, p. 73.
- ^ IPCC 2014, p. 616.
- ^ “Biofuels explained: Ethanol”. US Energy Information Administration. 18 June 2020. Archived from the original on 14 May 2021. Retrieved 16 May 2021.
- ^ Foley, Jonathan (5 March 2013). “It’s Time to Rethink America’s Corn System”. Scientific American. Archived from the original on 3 January 2020. Retrieved 16 May 2021.
- ^ Ayompe, Lacour M.; Schaafsma, M.; Egoh, Benis N. (January 2021). “Towards sustainable palm oil production: The positive and negative impacts on ecosystem services and human wellbeing”. Journal of Cleaner Production. 278: 123914. Bibcode:2021JCPro.27823914A. doi:10.1016/j.jclepro.2020.123914.
- ^ Lustgarten, Abrahm (20 November 2018). “Palm Oil Was Supposed to Help Save the Planet. Instead It Unleashed a Catastrophe”. The New York Times. Archived from the original on 17 May 2019. Retrieved 15 May 2019.
- ^ Smil 2017a, p. 161.
- ^ National Academies of Sciences, Engineering, and Medicine 2019, p. 3.
- ^ REN21 2021, pp. 113–116.
- ^ “The Role of Gas: Key Findings”. IEA. July 2019. Archived from the original on 1 September 2019. Retrieved 4 October 2019.
- ^ “Natural gas and the environment”. US Energy Information Administration. Archived from the original on 2 April 2021. Retrieved 28 March 2021.
- ^ Jump up to:a b Storrow, Benjamin. “Methane Leaks Erase Some of the Climate Benefits of Natural Gas”. Scientific American. Retrieved 31 May 2023.
- ^ Plumer, Brad (26 June 2019). “As Coal Fades in the U.S., Natural Gas Becomes the Climate Battleground”. The New York Times. Archived from the original on 23 September 2019. Retrieved 4 October 2019.
- ^ Gürsan, C.; de Gooyert, V. (March 2021). “The systemic impact of a transition fuel: Does natural gas help or hinder the energy transition?”. Renewable and Sustainable Energy Reviews. 138: 110552. Bibcode:2021RSERv.13810552G. doi:10.1016/j.rser.2020.110552. hdl:2066/228782.
- ^ Budinis, Sara; Krevor, Samuel; Dowell, Niall Mac; Brandon, Nigel; Hawkes, Adam (November 2018). “An assessment of CCS costs, barriers and potential”. Energy Strategy Reviews. 22: 61–81. Bibcode:2018EneSR..22…61B. doi:10.1016/j.esr.2018.08.003.
- ^ “Zero-emission carbon capture and storage in power plants using higher capture rates”. IEA. 7 January 2021. Archived from the original on 30 March 2021. Retrieved 14 March 2021.
- ^ Jump up to:a b Ritchie, Hannah (10 February 2020). “What are the safest and cleanest sources of energy?”. Our World in Data. Archived from the original on 29 November 2020. Retrieved 14 March 2021.
- ^ Evans, Simon (8 December 2017). “Solar, wind and nuclear have ‘amazingly low’ carbon footprints, study finds”. Carbon Brief. Archived from the original on 16 March 2021. Retrieved 15 March 2021.
- ^ IPCC 2018, 5.4.1.2.
- ^ IPCC AR6 WG3 2022, p. 38.
- ^ “Global Status Report 2024”. Global CCS Institute. pp. 57–58. Retrieved 19 October 2024. The report lists 50 facilities, of which 3 are direct air capture facilities and 3 are transport/storage facilities
- ^ Roser, Max (10 December 2020). “The world’s energy problem”. Our World in Data. Archived from the original on 21 July 2021. Retrieved 21 July 2021.
- ^ Rhodes, Richard (19 July 2018). “Why Nuclear Power Must Be Part of the Energy Solution”. Yale Environment 360. Yale School of the Environment. Archived from the original on 9 August 2021. Retrieved 24 July 2021.
- ^ “Nuclear Power in the World Today”. World Nuclear Association. June 2021. Archived from the original on 16 July 2021. Retrieved 19 July 2021.
- ^ Bailey, Ronald (10 May 2023). “New study: Nuclear power is humanity’s greenest energy option”. Reason.com. Retrieved 22 May 2023.
- ^ Ritchie, Hannah; Roser, Max (2020). “Nuclear Energy”. Our World in Data. Archived from the original on 20 July 2021. Retrieved 19 July 2021.
- ^ MacKay 2008, p. 162.
- ^ Jump up to:a b c d e Gill, Matthew; Livens, Francis; Peakman, Aiden (2014). “Nuclear Fission”. Future Energy. pp. 135–149. doi:10.1016/B978-0-08-102886-5.00007-4. ISBN 978-0-08-102886-5.
- ^ Muellner, Nikolaus; Arnold, Nikolaus; Gufler, Klaus; Kromp, Wolfgang; Renneberg, Wolfgang; Liebert, Wolfgang (August 2021). “Nuclear energy – The solution to climate change?”. Energy Policy. 155: 112363. Bibcode:2021EnPol.15512363M. doi:10.1016/j.enpol.2021.112363.
- ^ IPCC 2018, 2.4.2.1.
- ^ Timmer, John (21 November 2020). “Why are nuclear plants so expensive? Safety’s only part of the story”. Ars Technica. Archived from the original on 28 April 2021. Retrieved 17 March 2021.
- ^ Technical assessment of nuclear energy with respect to the ‘do no significant harm’ criteria of Regulation (EU) 2020/852 (‘Taxonomy Regulation’) (PDF) (Report). European Commission Joint Research Centre. 2021. p. 53. Archived (PDF) from the original on 26 April 2021.
- ^ Locatelli, Giorgio; Mignacca, Benito (2020). “Small Modular Nuclear Reactors”. Future Energy. pp. 151–169. doi:10.1016/B978-0-08-102886-5.00008-6. ISBN 978-0-08-102886-5.
- ^ McGrath, Matt (6 November 2019). “Nuclear fusion is ‘a question of when, not if'”. BBC. Archived from the original on 25 January 2021. Retrieved 13 February 2021.
- ^ Amos, Jonathan (9 February 2022). “Major breakthrough on nuclear fusion energy”. BBC. Archived from the original on 1 March 2022. Retrieved 10 February 2022.
- ^ “Energy Transition Investment Now On Par with Fossil Fuel”. Bloomberg NEF (New Energy Finance). 10 February 2023. Archived from the original on 27 March 2023.
- ^ Jaccard 2020, pp. 202–203, Chapter 11 – “Renewables Have Won”.
- ^ Jump up to:a b c d IPCC 2014, 7.11.3.
- ^ IEA 2021, pp. 106–110.
- ^ Jump up to:a b Evans, Simon; Gabbatiss, Josh (30 November 2020). “In-depth Q&A: Does the world need hydrogen to solve climate change?”. Carbon Brief. Archived from the original on 1 December 2020. Retrieved 1 December 2020.
- ^ Jaccard 2020, p. 203, Chapter 11 – “Renewables Have Won”.
- ^ “Reaching net zero emissions demands faster innovation, but we’ve already come a long way – Analysis”. International Energy Agency. 13 November 2023. Retrieved 30 April 2024.
- ^ Jump up to:a b IEA 2021, p. 15.
- ^ “Innovation – Energy System”. International Energy Agency. Retrieved 30 April 2024.
- ^ World Health Organization 2018, Executive Summary.
- ^ Vandyck, T.; Keramidas, K.; Kitous, A.; Spadaro, J.V.; et al. (2018). “Air quality co-benefits for human health and agriculture counterbalance costs to meet Paris Agreement pledges”. Nature Communications. 9 (1): 4939. Bibcode:2018NatCo…9.4939V. doi:10.1038/s41467-018-06885-9. PMC 6250710. PMID 30467311.
- ^ Jump up to:a b c d United Nations Environment Programme 2019, pp. 46–55.
- ^ IPCC 2018, p. 97.
- ^ Hopwood, David (May 2007). “Blueprint for sustainability?”. Refocus. 8 (3): 54–57. doi:10.1016/S1471-0846(07)70068-9.
- ^ United Nations Environment Programme 2019, p. 47.
- ^ “Introduction to System Integration of Renewables”. IEA. Archived from the original on 15 May 2020. Retrieved 30 May 2020.
- ^ Jump up to:a b c d Blanco, Herib; Faaij, André (January 2018). “A review at the role of storage in energy systems with a focus on Power to Gas and long-term storage” (PDF). Renewable and Sustainable Energy Reviews. 81: 1049–1086. Bibcode:2018RSERv..81.1049B. doi:10.1016/j.rser.2017.07.062.
- ^ REN21 2020, p. 177.
- ^ Bloess, Andreas; Schill, Wolf-Peter; Zerrahn, Alexander (February 2018). “Power-to-heat for renewable energy integration: A review of technologies, modeling approaches, and flexibility potentials”. Applied Energy. 212: 1611–1626. Bibcode:2018ApEn..212.1611B. doi:10.1016/j.apenergy.2017.12.073. hdl:10419/200120.
- ^ IEA 2020, p. 109.
- ^ Jump up to:a b Koohi-Fayegh, S.; Rosen, M.A. (February 2020). “A review of energy storage types, applications and recent developments”. Journal of Energy Storage. 27: 101047. Bibcode:2020JEnSt..2701047K. doi:10.1016/j.est.2019.101047.
- ^ Katz, Cheryl (17 December 2020). “The batteries that could make fossil fuels obsolete”. BBC. Archived from the original on 11 January 2021. Retrieved 10 January 2021.
- ^ Jump up to:a b “Climate change and batteries: the search for future power storage solutions” (PDF). Climate change: science and solutions. The Royal Society. 19 May 2021. Archived from the original on 16 October 2021. Retrieved 15 October 2021.
- ^ Hunt, Julian D.; Byers, Edward; Wada, Yoshihide; Parkinson, Simon; Gernaat, David E. H. J.; Langan, Simon; van Vuuren, Detlef P.; Riahi, Keywan (19 February 2020). “Global resource potential of seasonal pumped hydropower storage for energy and water storage”. Nature Communications. 11 (1): 947. Bibcode:2020NatCo..11..947H. doi:10.1038/s41467-020-14555-y. PMC 7031375. PMID 32075965.
- ^ Balaraman, Kavya (12 October 2020). “To batteries and beyond: With seasonal storage potential, hydrogen offers ‘a different ballgame entirely'”. Utility Dive. Archived from the original on 18 January 2021. Retrieved 10 January 2021.
- ^ Cole, Laura (15 November 2020). “How to cut carbon out of your heating”. BBC. Archived from the original on 27 August 2021. Retrieved 31 August 2021.
- ^ Ritchie, Hannah; Roser, Max (2020). “Electricity Mix”. Our World in Data. Archived from the original on 13 October 2021. Retrieved 16 October 2021.
- ^ IPCC 2018, 2.4.2.2.
- ^ IEA 2021, pp. 167–169.
- ^ United Nations Development Programme 2016, p. 30.
- ^ Jump up to:a b c Herrington, Richard (24 May 2021). “Mining our green future”. Nature Reviews Materials. 6 (6): 456–458. Bibcode:2021NatRM…6..456H. doi:10.1038/s41578-021-00325-9.
- ^ Mudd, Gavin M. “Metals and Elements Needed to Support Future Energy Systems”. In Letcher (2020), pp. 723–724.
- ^ Babbitt, Callie W. (August 2020). “Sustainability perspectives on lithium-ion batteries”. Clean Technologies and Environmental Policy. 22 (6): 1213–1214. Bibcode:2020CTEP…22.1213B. doi:10.1007/s10098-020-01890-3.
- ^ Jump up to:a b c IPCC AR6 WG3 2022, pp. 91–92.
- ^ Evans, Simon; Gabbatiss, Josh (30 November 2020). “In-depth Q&A: Does the world need hydrogen to solve climate change?”. Carbon Brief. Archived from the original on 1 December 2020. Retrieved 1 December 2020.
- ^ Jump up to:a b c Lewis, Alastair C. (10 June 2021). “Optimising air quality co-benefits in a hydrogen economy: a case for hydrogen-specific standards for NO x emissions”. Environmental Science: Atmospheres. 1 (5): 201–207. Bibcode:2021ESAt….1..201L. doi:10.1039/D1EA00037C. This article incorporates text from this source, which is available under the CC BY 3.0 license.
- ^ Reed, Stanley; Ewing, Jack (13 July 2021). “Hydrogen Is One Answer to Climate Change. Getting It Is the Hard Part”. The New York Times. Archived from the original on 14 July 2021. Retrieved 14 July 2021.
- ^ IRENA 2019, p. 9.
- ^ Bonheure, Mike; Vandewalle, Laurien A.; Marin, Guy B.; Van Geem, Kevin M. (March 2021). “Dream or Reality? Electrification of the Chemical Process Industries”. CEP Magazine. American Institute of Chemical Engineers. Archived from the original on 17 July 2021. Retrieved 6 July 2021.
- ^ Jump up to:a b Griffiths, Steve; Sovacool, Benjamin K.; Kim, Jinsoo; Bazilian, Morgan; Uratani, Joao M. (October 2021). “Industrial decarbonization via hydrogen: A critical and systematic review of developments, socio-technical systems and policy options”. Energy Research & Social Science. 80: 102208. doi:10.1016/j.erss.2021.102208.
- ^ Palys, Matthew J.; Daoutidis, Prodromos (May 2020). “Using hydrogen and ammonia for renewable energy storage: A geographically comprehensive techno-economic study”. Computers & Chemical Engineering. 136: 106785. doi:10.1016/j.compchemeng.2020.106785.
- ^ IRENA 2021, pp. 12, 22.
- ^ IEA 2021, pp. 15, 75–76.
- ^ Kjellberg-Motton, Brendan (7 February 2022). “Steel decarbonisation gathers speed | Argus Media”. www.argusmedia.com. Retrieved 7 September 2023.
- ^ Blank, Thomas; Molly, Patrick (January 2020). “Hydrogen’s Decarbonization Impact for Industry” (PDF). Rocky Mountain Institute. pp. 2, 7, 8. Archived (PDF) from the original on 22 September 2020.
- ^ Plötz, Patrick (31 January 2022). “Hydrogen technology is unlikely to play a major role in sustainable road transport”. Nature Electronics. 5 (1): 8–10. doi:10.1038/s41928-021-00706-6.
- ^ Fraser, Simon D.S.; Lock, Karen (December 2011). “Cycling for transport and public health: a systematic review of the effect of the environment on cycling”. European Journal of Public Health. 21 (6): 738–743. doi:10.1093/eurpub/ckq145. PMID 20929903.
- ^ “Global Greenhouse Gas Emissions Data”. United States Environmental Protection Agency. 12 January 2016. Archived from the original on 5 December 2019. Retrieved 15 October 2021.
- ^ Bigazzi, Alexander (May 2019). “Comparison of marginal and average emission factors for passenger transportation modes”. Applied Energy. 242: 1460–1466. Bibcode:2019ApEn..242.1460B. doi:10.1016/j.apenergy.2019.03.172.
- ^ Schäfer, Andreas W.; Yeh, Sonia (20 April 2020). “A holistic analysis of passenger travel energy and greenhouse gas intensities”. Nature Sustainability. 3 (6): 459–462. Bibcode:2020NatSu…3..459S. doi:10.1038/s41893-020-0514-9.
- ^ United Nations Environment Programme 2020, p. xxv.
- ^ IEA 2021, p. 137.
- ^ Pucher, John; Buehler, Ralph (2 November 2017). “Cycling towards a more sustainable transport future”. Transport Reviews. 37 (6): 689–694. doi:10.1080/01441647.2017.1340234.
- ^ Smith, John (22 September 2016). “Sustainable transport”. European Commission. Archived from the original on 22 October 2021. Retrieved 22 October 2021.
- ^ Knobloch, Florian; Hanssen, Steef V.; Lam, Aileen; Pollitt, Hector; Salas, Pablo; Chewpreecha, Unnada; Huijbregts, Mark A. J.; Mercure, Jean-Francois (23 March 2020). “Net emission reductions from electric cars and heat pumps in 59 world regions over time”. Nature Sustainability. 3 (6): 437–447. Bibcode:2020NatSu…3..437K. doi:10.1038/s41893-020-0488-7. PMC 7308170. PMID 32572385.
- ^ Bogdanov, Dmitrii; Farfan, Javier; Sadovskaia, Kristina; Aghahosseini, Arman; Child, Michael; Gulagi, Ashish; Oyewo, Ayobami Solomon; de Souza Noel Simas Barbosa, Larissa; Breyer, Christian (6 March 2019). “Radical transformation pathway towards sustainable electricity via evolutionary steps”. Nature Communications. 10 (1). doi:10.1038/s41467-019-08855-1. PMC 6403340. PMID 30842423.
- ^ Martini, Giorgio; Grigoratos, Theodoros (2014). Non-exhaust traffic related emissions – Brake and tyre wear PM. EUR 26648. Publications Office of the European Union. p. 42. ISBN 978-92-79-38303-8. OCLC 1044281650. Archived from the original on 30 July 2021.
- ^ Non-exhaust Particulate Emissions from Road Transport. 2020. pp. 8–9. doi:10.1787/4a4dc6ca-en. ISBN 978-92-64-45244-2.
- ^ “CO2 performance of new passenger cars in Europe”. www.eea.europa.eu. Retrieved 19 October 2022.
- ^ IEA 2021, pp. 133–137.
- ^ “Rail and waterborne – best for low-carbon motorised transport”. European Environment Agency. Archived from the original on 9 October 2021. Retrieved 15 October 2021.
- ^ Miller, Joe (9 September 2020). “Hydrogen takes a back seat to electric for passenger vehicles”. Financial Times. Archived from the original on 20 September 2020. Retrieved 9 September 2020.
- ^ IEA 2021, pp. 136, 139.
- ^ Biomass in a low-carbon economy (Report). UK Committee on Climate Change. November 2018. p. 18. Archived from the original on 28 December 2019. Retrieved 28 December 2019.
- ^ “Buildings”. IEA. Archived from the original on 14 October 2021. Retrieved 15 October 2021.
- ^ Mortensen, Anders Winther; Mathiesen, Brian Vad; Hansen, Anders Bavnhøj; Pedersen, Sigurd Lauge; Grandal, Rune Duban; Wenzel, Henrik (October 2020). “The role of electrification and hydrogen in breaking the biomass bottleneck of the renewable energy system – A study on the Danish energy system”. Applied Energy. 275: 115331. Bibcode:2020ApEn..27515331M. doi:10.1016/j.apenergy.2020.115331.
- ^ Knobloch, Florian; Pollitt, Hector; Chewpreecha, Unnada; Daioglou, Vassilis; Mercure, Jean-Francois (February 2019). “Simulating the deep decarbonisation of residential heating for limiting global warming to 1.5 °C”. Energy Efficiency. 12 (2): 521–550. arXiv:1710.11019. Bibcode:2019EnEff..12..521K. doi:10.1007/s12053-018-9710-0.
- ^ Alva, Guruprasad; Lin, Yaxue; Fang, Guiyin (February 2018). “An overview of thermal energy storage systems”. Energy. 144: 341–378. Bibcode:2018Ene…144..341A. doi:10.1016/j.energy.2017.12.037.
- ^ Plumer, Brad (30 June 2021). “Are ‘Heat Pumps’ the Answer to Heat Waves? Some Cities Think So”. The New York Times. Archived from the original on 10 September 2021. Retrieved 11 September 2021.
- ^ Abergel, Thibaut (June 2020). “Heat Pumps”. IEA. Archived from the original on 3 March 2021. Retrieved 12 April 2021.
- ^ Buffa, Simone; Cozzini, Marco; D’Antoni, Matteo; Baratieri, Marco; et al. (2019). “5th generation district heating and cooling systems: A review of existing cases in Europe”. Renewable and Sustainable Energy Reviews. 104: 504–522. Bibcode:2019RSERv.104..504B. doi:10.1016/j.rser.2018.12.059.
- ^ Lund, Henrik; Werner, Sven; Wiltshire, Robin; Svendsen, Svend; et al. (2014). “4th Generation District Heating (4GDH)”. Energy. 68: 1–11. doi:10.1016/j.energy.2014.02.089. Archived from the original on 7 March 2021. Retrieved 13 June 2021.
- ^ Abdolhamidi, Shervin (27 September 2018). “An ancient engineering feat that harnessed the wind”. BBC. Archived from the original on 12 August 2021. Retrieved 12 August 2021.
- ^ “How cities are using nature to keep heatwaves at bay”. United Nations Environment Programme. 22 July 2020. Archived from the original on 11 September 2021. Retrieved 11 September 2021.
- ^ Jump up to:a b “Four Things You Should Know About Sustainable Cooling”. World Bank. 23 May 2019. Archived from the original on 11 September 2021. Retrieved 11 September 2021.
- ^ Mastrucci, Alessio; Byers, Edward; Pachauri, Shonali; Rao, Narasimha D. (March 2019). “Improving the SDG energy poverty targets: Residential cooling needs in the Global South”. Energy and Buildings. 186: 405–415. Bibcode:2019EneBu.186..405M. doi:10.1016/j.enbuild.2019.01.015.
- ^ Jump up to:a b c Smith & Pillarisetti 2017, pp. 145–146.
- ^ “Cooking appliances”. Natural Resources Canada. 16 January 2013. Archived from the original on 30 July 2021. Retrieved 30 July 2021.
- ^ World Health Organization; International Energy Agency; Global Alliance for Clean Cookstoves; United Nations Development Programme; Energising Development; and World Bank (2018). Accelerating SDG 7 Achievement Policy Brief 02: Achieving Universal Access to Clean and Modern Cooking Fuels, Technologies and Services (PDF) (Report). United Nations. p. 3. Archived (PDF) from the original on 18 March 2021.
- ^ World Health Organization 2016, p. 75.
- ^ IPCC 2014, p. 29.
- ^ World Health Organization 2016, p. 12.
- ^ REN21 2020, p. 40.
- ^ IEA 2020, p. 135.
- ^ United Nations Environment Programme 2019, p. 50.
- ^ Åhman, Max; Nilsson, Lars J.; Johansson, Bengt (4 July 2017). “Global climate policy and deep decarbonization of energy-intensive industries”. Climate Policy. 17 (5): 634–649. Bibcode:2017CliPo..17..634A. doi:10.1080/14693062.2016.1167009.
- ^ United Nations Environment Programme 2019, p. xxiii.
- ^ IEA 2021, p. 186.
- ^ Jump up to:a b c United Nations Environment Programme 2019, pp. 39–45.
- ^ Jaccard 2020, p. 109, Chapter 6 – We Must Price Carbon Emissions”.
- ^ Jump up to:a b United Nations Environment Programme 2019, pp. 28–36.
- ^ Ciucci, M. (February 2020). “Renewable Energy”. European Parliament. Archived from the original on 4 June 2020. Retrieved 3 June 2020.
- ^ “State Renewable Portfolio Standards and Goals”. National Conference of State Legislators. 17 April 2020. Archived from the original on 3 June 2020. Retrieved 3 June 2020.
- ^ IEA 2021, pp. 14–25.
- ^ IEA 2021, pp. 184–187.
- ^ IEA 2021, p. 16.
- ^ Jaccard 2020, pp. 106–109, Chapter 6 – “We Must Price Carbon Emissions”.
- ^ Plumer, Brad (8 October 2018). “New U.N. Climate Report Says Put a High Price on Carbon”. The New York Times. Archived from the original on 27 September 2019. Retrieved 4 October 2019.
- ^ Green, Jessica F (April 2021). “Does carbon pricing reduce emissions? A review of ex-post analyses”. Environmental Research Letters. 16 (4): 043004. Bibcode:2021ERL….16d3004G. doi:10.1088/1748-9326/abdae9.
- ^ IPCC 2018, 2.5.2.1.
- ^ State and Trends of Carbon Pricing 2019 (PDF) (Report). World Bank. June 2019. pp. 8–11. doi:10.1596/978-1-4648-1435-8. hdl:10986/29687. ISBN 978-1-4648-1435-8. Archived (PDF) from the original on 6 May 2020.
- ^ “Revenue-Neutral Carbon Tax | Canada”. United Nations Framework Convention on Climate Change. Archived from the original on 28 October 2019. Retrieved 28 October 2019.
- ^ Carr, Mathew (10 October 2018). “How High Does Carbon Need to Be? Somewhere From $20–$27,000”. Bloomberg. Archived from the original on 5 August 2019. Retrieved 4 October 2019.
- ^ “EAC launches new inquiry weighing up carbon border tax measures”. UK Parliament. 24 September 2021. Archived from the original on 24 September 2021. Retrieved 14 October 2021.
- ^ Plumer, Brad (14 July 2021). “Europe Is Proposing a Border Carbon Tax. What Is It and How Will It Work?”. The New York Times. Archived from the original on 10 September 2021. Retrieved 10 September 2021.
- ^ Bharti, Bianca (12 August 2021). “Taxing imports of heavy carbon emitters is gaining momentum – and it could hurt Canadian industry: Report”. Financial Post. Archived from the original on 3 October 2021. Retrieved 3 October 2021.
- ^ United Nations Environment Programme 2020, p. vii.
- ^ IEA 2021, p. 13.
- ^ IEA 2021, pp. 14–18.
- ^ IRENA, IEA & REN21 2018, p. 19.
- ^ Jump up to:a b “24 million jobs to open up in the green economy”. International Labour Organization. 14 May 2018. Archived from the original on 2 June 2021. Retrieved 30 May 2021.
- ^ Catsaros, Oktavia (26 January 2023). “Global Low-Carbon Energy Technology Investment Surges Past $1 Trillion for the First Time”. Bloomberg NEF (New Energy Finance). Figure 1. Archived from the original on 22 May 2023.
Defying supply chain disruptions and macroeconomic headwinds, 2022 energy transition investment jumped 31% to draw level with fossil fuels
- ^ “Global Clean Energy Investment Jumps 17%, Hits $1.8 Trillion in 2023, According to BloombergNEF Report”. BNEF.com. Bloomberg NEF. 30 January 2024. Archived from the original on 28 June 2024.
Start years differ by sector but all sectors are present from 2020 onwards.
- ^ Jump up to:a b 2024 data: “Energy Transition Investment Trends 2025 / Abridged report” (PDF). BloombergNEF. 30 January 2025. p. 9. Archived (PDF) from the original on 2 February 2025.
- ^ Jump up to:a b Mazzucato, Mariana; Semieniuk, Gregor (February 2018). “Financing renewable energy: Who is financing what and why it matters”. Technological Forecasting and Social Change. 127: 8–22. doi:10.1016/j.techfore.2017.05.021.
- ^ United Nations Development Programme & United Nations Framework Convention on Climate Change 2019, p. 24.
- ^ IPCC 2018, p. 96.
- ^ IEA, IRENA, United Nations Statistics Division, World Bank, World Health Organization 2021, pp. 129, 132.
- ^ United Nations Framework Convention on Climate Change 2018, p. 54.
- ^ United Nations Framework Convention on Climate Change 2018, p. 9.
- ^ Roberts, J. Timmons; Weikmans, Romain; Robinson, Stacy-ann; Ciplet, David; Khan, Mizan; Falzon, Danielle (March 2021). “Rebooting a failed promise of climate finance”. Nature Climate Change. 11 (3): 180–182. Bibcode:2021NatCC..11..180R. doi:10.1038/s41558-021-00990-2.
- ^ Radwanski, Adam (29 September 2021). “Opinion: As pivotal climate summit approaches, Canada at centre of efforts to repair broken trust among poorer countries”. The Globe and Mail. Archived from the original on 30 September 2021. Retrieved 30 September 2021.
- ^ “Here are the clean energy innovations that will beat climate change”. European Investment Bank. Retrieved 26 September 2022.
- ^ “Home”. www.oecd-ilibrary.org. Retrieved 19 October 2022.
- ^ Bridle, Richard; Sharma, Shruti; Mostafa, Mostafa; Geddes, Anna (June 2019). “Fossil Fuel to Clean Energy Subsidy Swaps: How to pay for an energy revolution” (PDF). International Institute for Sustainable Development. p. iv. Archived (PDF) from the original on 17 November 2019.
- ^ Watts, Nick; Amann, Markus; Arnell, Nigel; Ayeb-Karlsson, Sonja; Belesova, Kristine; Boykoff, Maxwell; Byass, Peter; Cai, Wenjia; Campbell-Lendrum, Diarmid; Capstick, Stuart; Chambers, Jonathan; Dalin, Carole; Daly, Meaghan; Dasandi, Niheer; Davies, Michael; Drummond, Paul; Dubrow, Robert; Ebi, Kristie L; Eckelman, Matthew; Ekins, Paul; Escobar, Luis E; Fernandez Montoya, Lucia; Georgeson, Lucien; Graham, Hilary; Haggar, Paul; Hamilton, Ian; Hartinger, Stella; Hess, Jeremy; Kelman, Ilan; Kiesewetter, Gregor; Kjellstrom, Tord; Kniveton, Dominic; Lemke, Bruno; Liu, Yang; Lott, Melissa; Lowe, Rachel; Sewe, Maquins Odhiambo; Martinez-Urtaza, Jaime; Maslin, Mark; McAllister, Lucy; McGushin, Alice; Jankin Mikhaylov, Slava; Milner, James; Moradi-Lakeh, Maziar; Morrissey, Karyn; Murray, Kris; Munzert, Simon; Nilsson, Maria; Neville, Tara; Oreszczyn, Tadj; Owfi, Fereidoon; Pearman, Olivia; Pencheon, David; Phung, Dung; Pye, Steve; Quinn, Ruth; Rabbaniha, Mahnaz; Robinson, Elizabeth; Rocklöv, Joacim; Semenza, Jan C; Sherman, Jodi; Shumake-Guillemot, Joy; Tabatabaei, Meisam; Taylor, Jonathon; Trinanes, Joaquin; Wilkinson, Paul; Costello, Anthony; Gong, Peng; Montgomery, Hugh (November 2019). “The 2019 report of The Lancet Countdown on health and climate change: ensuring that the health of a child born today is not defined by a changing climate”. The Lancet. 394 (10211): 1836–1878. Bibcode:2019Lanc..394.1836W. doi:10.1016/S0140-6736(19)32596-6. PMC 7616843. PMID 31733928.
- ^ United Nations Development Programme 2020, p. 10.
- ^ Kuzemko, Caroline; Bradshaw, Michael; Bridge, Gavin; Goldthau, Andreas; Jewell, Jessica; Overland, Indra; Scholten, Daniel; Van de Graaf, Thijs; Westphal, Kirsten (October 2020). “Covid-19 and the politics of sustainable energy transitions”. Energy Research & Social Science. 68: 101685. Bibcode:2020ERSS…6801685K. doi:10.1016/j.erss.2020.101685. PMC 7330551. PMID 32839704.
- ^ IRENA 2021, p. 5.